The Paris Accords are arguably the most important initiative ever undertaken to address globally the future of our environment and the future of energy in the 21st century, and beyond. What are the Accords? In short, 196 countries signed on to reduce their anthropogenic gas output “as soon as possible” in order to limit global warming to “well below 2 degrees C” (3.6°F) above pre-industrial levels. That is, by 2030 emissions would need to be cut by about 50 percent.1 However, it’s not as clear-cut as it may seem. First, the agreement does not include international aviation or shipping, which require massive use of fossil fuels. Perhaps more importantly, many environmentalists have noted that the Accords do not bind any of the signatories to the reduction of greenhouse gasses. The eye-opening section of the Accords states that countries must make an “ambitious effort” towards “achieving the purpose of the Agreement.” And every five years, each country must put forth a more ambitious strategy than the previous five years. However, there is absolutely no process to force signatories to mitigate their release of anthropogenic gases.
While some countries, such as the U.S., made unconditional pledges to reduce anthropogenic gases, many others, e.g., India, refused to sign the Accords unless they obtained technical and financial aid. As a result, industrialized countries agreed to contribute $100 billion per year by 2020 to the Green Climate Fund (GCF) and other ventures to help less developed countries. Each industrialized country determines how much money it should contribute to the fund.2 Paradoxically, however, the GCF refused to ban the use of the funds to support fossil fuel projects such as coal power plants.
As of July 31, 2020, the fund raised just over ten billion dollars. The United States has given twice as much as any other country (three billion dollars). Interestingly, China, the second richest nation in the world, was designated as a developing country with no obligation to commit funds, leaving mainly the United States and Europe, along with a few rich Asian countries, to fund the GCF. It should be noted that China has a market share in the solar panel supply chain of more than 80 percent,3 so the Paris Accords have proven a financial bonanza for that nation. Other countries, such as Iran, refused to ratify the Accords; Russia and North Korea have also not contributed based on their developing country status. Many researchers have emphasized that large increases in contributions will be needed if the Paris Accords are to achieve the decrease in anthropogenic gases that the body seeks.
Without fracking, the U.S. would still be the largest world importer of oil and gas.
Perhaps the only meaningful way to assess the impact of the Paris Accords is to look at the carbon dioxide emissions of various countries. The COVID-19 pandemic had an impact on all countries except China and Saudi Arabia (even COVID did not slow China’s high level of emissions). The United States has been decreasing emissions since 2007–2008. On the other hand, China’s increase has skyrocketed since the Paris Accords were signed in 2015. As many economists note, America is adversely impacting its economy while trying to transition to clean energy. China, however, and to a lesser extent India, have experienced no significant impact on their emissions, particularly in the last six years.
In game theory, the results from the Paris Accords exemplify what is called the tragedy of the commons. Participants (in this case, national leaders) will act in rational ways that serve their own best interests. Not surprisingly, most of the world’s ordinary people do not want to degrade their standard of living by rejecting cheap energy. As Matt Ridley succinctly stated the dilemma:
…so humankind faces a stark dilemma in the coming century between continuing a carbon-fueled prosperity until global warming brings it to a calamitous halt, or restricting the use of carbon and risking a steep decline in living standards because of the lack of alternative sources of energy that are cheap enough. Either prospect might be catastrophic.
So, what, realistically, can be done?
Fracking: An Interim Solution
There are three major fossil fuel contributors to the production of anthropogenic gases such as CO2: coal, natural gas (primarily methane), and petroleum. Of the three, coal is, by far, the worst polluter, followed by petroleum. Both contain various potentially serious pollutants, such as sulfur which can cause acid rain through sulfuric acid that forms in the atmosphere. Natural gas has some impurities, but they can be easily removed before it is burned. Natural gas also has a higher energy density—that is, it turns out more energy relative to an equivalent weight of coal or petroleum products, including ethanol derived from corn.
Since the beginning of fracking and the progressive replacement of other fossil fuels by natural gas, the United States has become the world leader in reducing its output of anthropogenic gas. The decrease in CO2 contamination since 1990 has been eight percent. Methane, also an anthropogenic gas, has been reduced by 17 percent over the same interval. As Gary Sernovitz concluded: “the United States has led the world in carbon dioxide emissions reduction because of shale gas [use of natural gas instead of coal and oil].”4
Note that, in addition to reducing emissions, the United States—not Saudi Arabia or Russia—has become the world’s largest producer of oil and gas by a large margin. That dominance came with the technology that enabled the United States’ producers to frack horizontally. The halcyon days of the 1960s, when the United States led production worldwide, gave way to the lean decades when the U.S. became dependent on oil imports to meet its energy needs. By the early 1980s, even secondary recovery processes in declining oil fields could not increase American production. That decline in oil production continued until about 2007, when fracking increased. By 2019, the U.S. was fully meeting all domestic oil and natural gas needs and even exporting, thus cutting dependence on the troubled Middle East and an increasingly hostile Russia. Without fracking, the U.S. would still be the largest world importer of oil and gas. Further, that increase in production has extensively replaced the need to burn coal.
How does fracking work?
Drilling for oil has long been a financially risky business. Most wells never produce a drop of oil. However, that changed to a great extent with the advent of gas and oil production through fracking. The new targets—usually oil shales—were discovered decades ago by previous drilling. They were ignored at that time because even though shales have an immense amount of void space between the tiny clay particles (highly porous), most of the void spaces are not interconnected (thus having poor permeability). Production companies need permeable rocks to produce oil and/or gas, or so it was thought.
Fracking sounds ominous and sinister and conjures up visions of rock being fractured all the way to potable water zones. Yet it is nothing of the sort. The technique took decades of testing and experimentation in wells to develop. The drill holes typically go down for thousands of feet below the surface and are protected with a cemented casing that has only been perforated in small sections, usually located at the bottom of the hole where the target rock exists. The secret is hydraulic pressure from fluids that are injected into the well that cause the shale to fracture. The fracturing is usually limited to about 300 feet in an outward radius around the drill hole where the cemented casing has been perforated.
Yet, one cannot emphasize enough the critical importance of protecting the water table when drilling. State and Federal regulations require a well to be sealed off at least 50 feet below where potable groundwater can be produced, and those laws have been in place as far back as anyone can remember. The drill pipe is tripped (pulled completely out of the hole) when regulators deem the surface casing should be set to protect the water table (usually, something on the order of 500 feet). The casing is cemented in place, and if that is done correctly, we know from the drilling of hundreds of thousands of wells over many decades that the water table is protected. After the surface casing is set, drilling is continued until the target zone is reached. The pipe is tripped again, and the entire well is generally set with cemented production casing. The hole is plugged at the bottom, usually up to 50 feet below the horizon of interest. The casing is then perforated by tools that blow holes in it precisely where the rock containing oil and/or gas exists.
To take advantage of fracking, horizontal drilling, another technological advance, was added to the arsenal of new production techniques. With the ability to target a bit within inches of a desired location, drillers learned how to gradually arc a pipe into the horizontal. The technology turned out to be a bonanza when combined with fracking. Companies drilled and set casing directly within and parallel to the oil shales enabling them to frack large sections of the rock that sent production through the ceiling.
The Risks
The chemicals used in fracking were originally a trade secret, but eventually companies published the composition of their fracking liquids. It turns out that 90 percent of the frack is made up of water, 9.5 percent consists of a proppant which is usually sand, and 0.5 percent consists of “scary” chemicals such as chloride, polyacrylamide, ethylene glycol, glutaraldehyde, etc., which have been the focus of arguments against the process. The target oil shales are typically thousands of feet below the surface (sometimes even miles) and if the casing is cemented properly, those chemicals stay completely out of harm’s way. The sand serves as a support to keep the fractures (caused by the pressurized fluid) propped open so gas and/or oil will flow (creating permeability). It takes a lot of water to frack a well. Estimates are that a typical frack uses between four and eight million gallons of water and about six million pounds of sand. Unfortunately, not all the fracking fluid stays in the hole. Some resurfaces. The water that comes back is reused or disposed of by pumping it into former producing fields in a concerted effort to make sure the chemicals within the water (even if they are only 0.5 percent) are sealed deep below the surface.
It has been widely reported that fracking causes earthquakes. However, fracking in itself is not responsible for the earthquakes. Rather, the disposal of water being pumped into the ground (usually from fracking) causes the seismic activity by reducing friction along faults. The typical increase in seismic activity in a state such as Oklahoma is usually mitigated effectively by diverting the injection of water from fields responsible for the activity or by requiring the water to be disposed of through other methods. In fact, the water has become so valuable that many companies reuse the water after it has been “cleaned.”
The manufacturing of one electric vehicle produces about 16 tons of CO2 compared to the 5 tons generated to make a gas-powered car.
In the early days of fracking some rogue drillers set poorly cemented casing that allowed gas to seep into the water table. The infamous water taps that caught fire in Dimock, Pennsylvania, were the result of poor casing in 27 holes. In 2015, the Environmental Protection Agency (EPA) produced a summary paper entitled Assessment of the Potential Impacts of Hydraulic Fracturing for Oil and Gas on Drinking Water Resources5 and concluded, “Assessment shows hydraulic fracturing activities have not led to widespread, systemic impacts to drinking water resources.” While the gas industry has made mistakes, subsequent regulations have abated those problems. It is reprehensible that some companies would not protect the water table at all costs; they should incur the penalties they received and be forced to make full restitution to those people they injured.
CLEAN ENERGY
Are solar and wind truly the next step?
After governments and private entities have spent hundreds of billions of dollars to subsidize clean energy, the experiment in the transition from fossil fuels has provided us with evidence that clean energy is not exactly the panacea many hoped for. Perhaps the most significant issue is that, because sunlight and wind are intermittent, their ability to supply clean electricity is not as reliable as other forms of energy. Those sources provide energy only 10–30 percent of the time during most of the year,6 leading to rolling blackouts in states such as California that are heavily dependent on clean energy. This makes storing energy one of the biggest clean energy dilemmas. The sun and the winds don’t always cooperate with our high-energy demands.
One suggestion has been to pump water uphill into reservoirs so that it can later be released over turbines when energy is needed. However, that solution is problematic, particularly in areas where water is scarce and land expensive. In addition, the energy required to pump water uphill along with the building of the infrastructure make that solution costly. Barring any better solutions, solar and wind farms have defaulted to using batteries to store energy during peak production. That, however, is not a realistic solution either: if we take the amount of energy Europe typically stores in fossil fuels for use in the winter, it would cost about 100 trillion dollars to store the same amount of energy in batteries. There is no doubt that, over time, batteries will become more efficient at storing energy, but batteries can’t violate the basic laws of physics, which render them wanting as a method of mass storage.7
Now that solar and wind have become important to the economy over the last two decades, replacing aging solar panels and turbines is becoming a serious problem in the form of electronic waste (e-waste). The International Renewable Energy Agency reports that by 2050 something on the order of 78 million metric tons of solar panels will need to be replaced and we will be dealing with six million metric tons of solar e-waste each year. Solar panels, depending on their type, contain valuable elements such as silver, aluminum, gallium, phosphorus, boron, palladium, copper, titanium, cadmium, tellurium, iridium, selenium, and of course, silica. Europe has recycling requirements, but the United States has no such nationwide laws, though some states require recycling. Unfortunately, many of the valuable rare elements are diluted when mixed with other compounds and separation is not economically efficient. Normally only copper, aluminum, and glass are recovered and valued at about $3 per solar panel. That sounds like a fair amount until you consider transportation costs to the refineries, which fall somewhere between $12 and $25 whereas sending a panel to a local landfill costs about a dollar. Fortunately, there are organizations such as the National Renewable Energy Laboratory that are assessing ways to recover all the elements in high purity.8 Only time will tell if any process will be economically feasible. Meanwhile, we face the loss of potentially valuable metal resources to landfills.
Solar and wind farms occupy immense areas that adversely impact wildlife habitats—wind turbines are killing millions of birds and bats,9 and when building the Ivanpah solar farm in California, the endangered desert tortoise population had to be relocated, killing some in the process. Wind farms also have environmental problems. A great deal of cement and iron go into the building of the turbine structures. The manufacturing of cement is one of the major contributors to greenhouse gasses, and iron has to be mined through gas-guzzling equipment.
Electric Vehicles
A major goal of the green revolution is to replace gas and diesel cars and trucks with electric vehicles. Because gas-powered cars do not require expensive rare metals and avoid the cost of mining those rare metals, manufacturing them is much cheaper than EVs. It is estimated to take about 25 barrels of oil or the equivalent in coal or natural gas to make one electric vehicle (much more than two times what it takes to make a gas-powered car). Put another way, the manufacturing of one electric vehicle produces about 16 tons of CO2 compared to the five tons generated to make a gas-powered car. Estimates suggest one would need to drive a new electric vehicle for 60,000 to 100,000 miles, depending on the size of the battery, before the EV overcomes the carbon dioxide output used to make the vehicle and the electricity still being generated from fossil fuels. As Mark Mills, a physicist with the Manhattan Institute, reminds us, the world has about 15–18 million electric vehicles. If that number rose to the aspirational level of, say, 500 million (approximately the number of cars in the U.S. and Europe), it would reduce oil production by about 10 percent. That is not insignificant, but it does not sound like a meaningful transition away from fossil fuels. Cheap fossil fuels are still the major source for the generation of the electricity that supplies electric cars.
Efforts are currently being made to demand the removal of all fossil fuel-driven cars by 2035. In California alone, the estimated 12.5 million electric vehicles added to the grid by 2035 will require two to three times more energy generated to keep up with the demand. California has made no plans to expand their grid by anything near this amount. Thus, it is highly unlikely California (or any other state) will be able to ramp up grids for electrical energy with solar and wind energy to meet the coming EV demand.10
Batteries, not only in electric cars but those used to store energy from solar and wind farms, present a number of additional, indirect problems. First, an electric vehicle needs 12–15 percent more energy than what is added to a recharged battery. Batteries are notoriously inefficient means of conserving energy because some of the energy leaks away (called transmission loss), some is converted to heat, and some keeps the battery from overheating.11 Second, the battery in an EV weighs about 1,000 pounds and can weigh as much as 3,000 pounds. Compare that to the 80-pound full gas tank it replaces, and it becomes clear that a lot more energy is required to drive an EV than a gas-powered car.12 The lithium-ion cells that are the mainstay of most battery use not only contain that element but also cobalt and various rare earth elements. One battery requires the mining of approximately 500,000 pounds of ore and rock. (Again, these are often located in hostile or unstable nations). Ironically, the environmental movement has pushed hard for decades to restrict mining through regulations. The resulting mining laws have made it virtually impossible to begin mining projects and make them economical in the U.S., even though high-grade ores are available. Consequently, those necessary metals are mined in countries with minimal environmental regulation and protection for their people. Cobalt is a good example. Cobalt is especially unfriendly to the environment:
Mining cobalt produces hazardous tailings and slags that can leach into the environment, and studies have found high exposure in nearby communities, especially among children, to cobalt and other metals. Extracting the metals from their ores also requires a process called smelting, which can emit sulfur oxide and other harmful air pollution.13
Something on the order of 70 percent of our cobalt comes from the Democratic Republic of Congo, where unregulated mining is often performed by subsistence miners and their children using archaic hand tools. As you might imagine, health and safety risks are afterthoughts under these conditions.
Lithium is mined primarily within the so-called “lithium triangle,” which covers sections of Argentina, Chile, and Bolivia. The salts are dissolved and pumped to the surface into huge evaporating ponds that concentrate the metal. To produce one ton of lithium, the process uses approximately 2.2 million liters of water, which can negatively affect local farming.
According to the Institute for Energy Research, the United States imports about 80 percent of its rare earth elements from China,14 which makes it highly dependent on what is increasingly becoming an adversary nation. Unfortunately, the recycling of batteries is still in its infant stages.
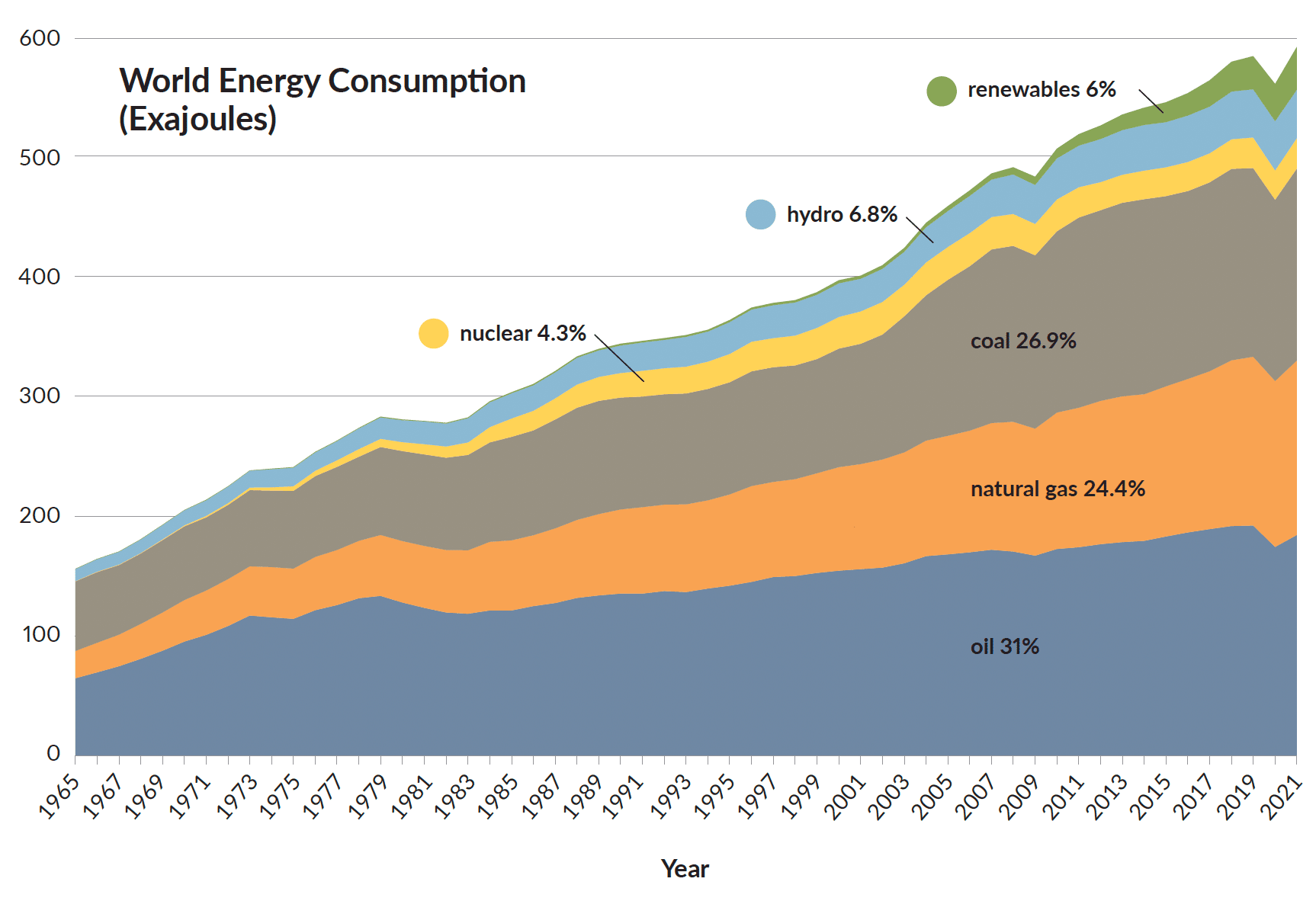
Figure 1. World energy consumption in exajoules of various commodities (BP Statistical View of World Energy, https://bit.ly/3AqaRuB). The percent of world consumption is based on 2021 usage. Renewable energy includes solar, wind, flowing water (excluding hydroelectric), geothermal, etc. Click graph to enlarge.
The Economics of Clean Energy
The world currently acquires about six percent of its total energy from renewable energy (see Figure 1). All current trends suggest that the major transition away from fossil fuels will be accomplished through the use of wind and solar along with battery storage. Yet, six percent can only mark the beginning of a true transition. Even in the United States, wind and solar only make up four percent of energy needs. What we have learned from renewables so far is that a real transition will be slow, difficult, and immensely expensive. The world has spent a staggering direct cost of approximately five trillion dollars over the past 15 years to reach the current renewable production. And another five trillion has probably been spent through indirect spending.15 Renewables have become cheaper (although prices are now increasing), but amping up to the stated goal of 70 percent renewables in the next 15–25 years is but an aspirational goal. The limited metal resources alone make that goal highly improbable. The IEA data demonstrate that replacing fossil fuel combustion would require a 2,000 to 7,000 percent increase in the tonnage of metals mined per unit of energy. The variation derives from the source of the clean energy. Offshore wind requires the highest percentage, while solar, onshore wind, and electric vehicles require less. Put another way, it would be the biggest increase in the supply of metals in all of human history, assuming it could be accomplished in the next two decades.
With the increase in demand for metals and the inability to supply the demand, prices will increase precipitously for the requisite metals and that, in turn, will greatly impact the cost of clean energy. Perhaps even more disturbing from a geopolitical perspective is that an increasingly confrontational China controls the refining of these metals and the mining of the rare earth elements.
California and Germany have been quite aggressive in the implementation of solar and wind energy and so serve as a testing ground for the economic feasibility of those sources. Californians paid almost 20 cents per kWh compared with about 11 cents per kWh country-wide in 2022. During the period, even though the price of solar and wind energy fell, Californians saw and felt energy prices explode. The land requirements and the unreliable nature of solar and wind required huge new costs related to storage and transmissions that must be addressed by those advocating for two trillion dollars in government spending (which must result in an increase in either taxes or, more likely, debt) to increase renewables, particularly wind and solar.16 Thus, the conundrum… How can we transition away from fossil fuels without destroying our economies?
Nuclear Energy
Wind, solar, and battery storage have had only a small impact on reducing our dependence on fossil fuels, even though we have spent massive sums of money to augment their use. As demonstrated, they are also detrimental to the environment, deplete valuable commodities of metals, and their potential as a major source of energy is limited.
It requires three million solar panels to replace one nuclear power plant, yet we continue to shut down our nuclear power plants and construct wind and solar farms.
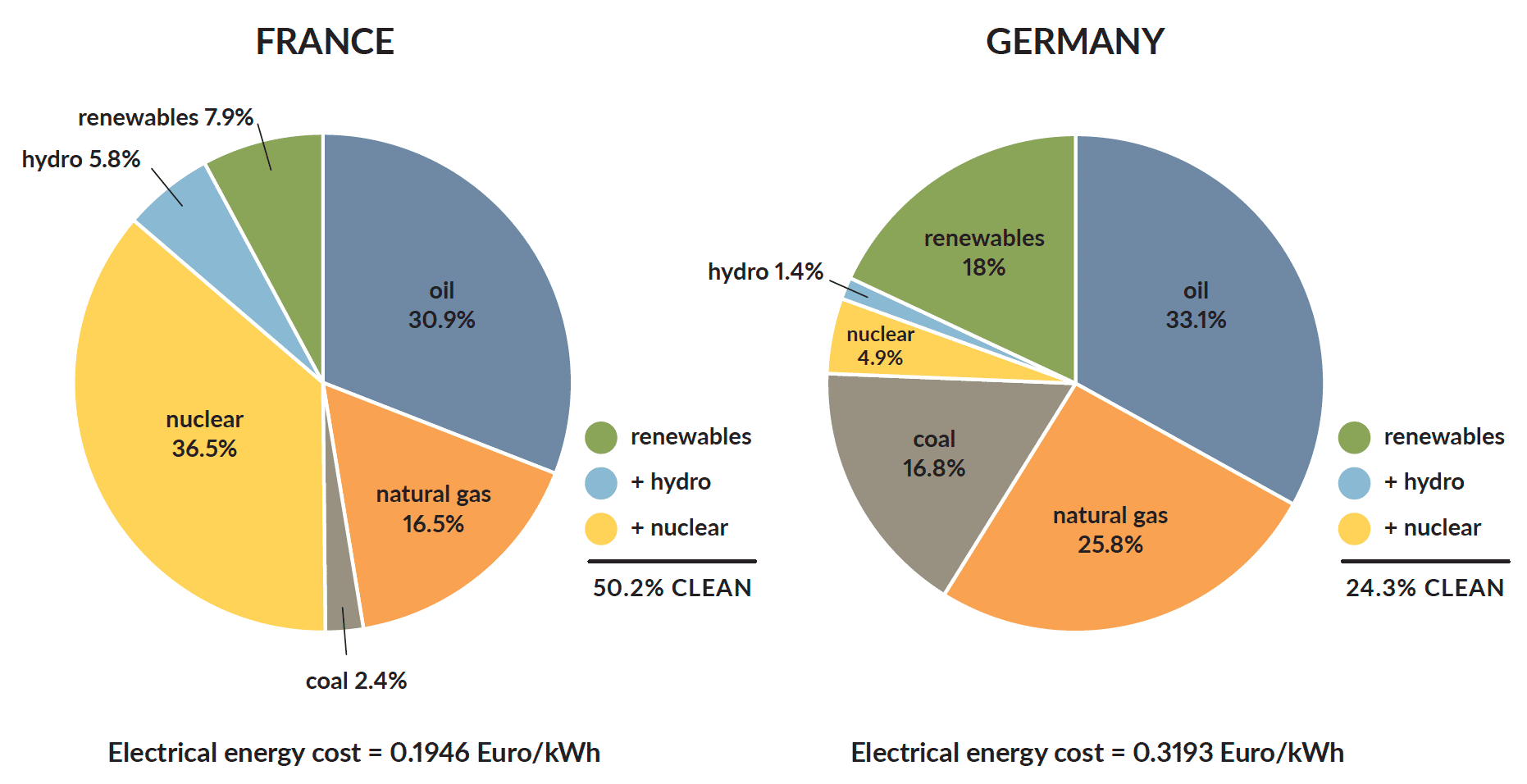
Figure 2. Comparison of energy consumption between France and Germany in percent (BP Statistical Review of World Energy, 2022, https://bit.ly/43X4dtI). Note the difference in electrical energy costs at the bottom of the diagram (kWh = kilowatt-hour; Eurostat database). Nuclear is considered clean energy in the diagram. Click chart to enlarge.
Compare 2022 energy usage in Germany and France (see Figure 2). Germany decided to move toward renewables, while France turned more toward nuclear energy. Coal usage in Germany has increased considerably due to the Ukraine War, but Germany still depends on a large amount of renewable energy for electricity (18 percent)—more than two times as much as France, which has only 7.9 percent renewables. Yet Germans pay twice as much for electricity as do the French. The difference is in the amount of nuclear energy (36.5 percent) that France uses to produce electricity. Nuclear makes France’s clean energy almost two times cheaper, primarily because, unlike wind and solar, it can be generated consistently. In April 2023, Germany shut down its last three remaining nuclear reactors.17
The World Nuclear Association has taken a hard look at the cost of nuclear energy and concluded: “system costs for nuclear power (as well as coal and gas-fired generation) are very much lower than for intermittent renewables.”18 Yet the world’s energy consumption of wind and solar has exploded compared to that of nuclear energy. Even Solar Reviews concludes:

This article appeared in Skeptic magazine 28.2
Buy print edition
Buy digital edition
Subscribe to print edition
Subscribe to digital edition
Download our app
Although building nuclear power plants has a high initial cost, it’s relatively cheap to produce energy from them and they have low operating costs. Also, nuclear power doesn’t experience the same kind of price fluctuations that traditional fossil fuel energy sources like coal and natural gas do [and solar and wind]. Because of that, the price of nuclear energy can be predicted well into the future.19
It requires three million solar panels to replace one nuclear power plant, yet we continue to shut down our nuclear power plants and construct wind and solar farms. At least 163 nuclear power plants were planned or under construction but canceled in the U.S.20 According to the Intergovernmental Panel on Climate Change (IPCC) data, nuclear energy generation produces four times less carbon pollution than solar farms. Consider also that wind and solar use more land and require more materials such as cement and steel and, of course, more mining of materials such as the rare metals compared to nuclear. Perhaps the most important advantage of nuclear power is its production of consistent energy. Nuclear power, according to the Department of Energy, is 92 percent efficient, whereas solar and wind are 26 and 37 percent efficient, respectively.21
Perhaps the key to an energy abundant future has been there before us all along. We just don’t seem to have chosen wisely.
About the Author
Marc J. Defant is a professor of geology at the University of South Florida. He specializes in the study of volcanoes, specifically the geochemistry of volcanic rocks. He has been funded by the NSF, National Geographic, the American Chemical Society, and the National Academy of Sciences and has published in many international journals, including Nature. He is the author of Voyage of Discovery: From the Big Bang to the Ice Age.
Disclosure
The author worked for Schlumberger Well Services and Shell Oil Company between 1977–1980.
References
- https://go.nature.com/3KS7Acl
- Secretariat, United Nations (2021). Nationally determined contributions under the Paris Agreement-Synthesis report by the secretariat. In Proceedings of the Conference of the Parties Serving as the Meeting of the Parties to the Paris Agreement Third Session (Vol. 31).
- https://bit.ly/3UOrWI2
- Sernovitz, G. (2016). The Green and the Black: The Complete Story of the Shale Revolution, the Fight Over Fracking, and the Future of Energy. St. Martin’s Press.
- https://bit.ly/3AfXywZ
- https://bit.ly/2WCFk2R
- https://bit.ly/3ot41lD
- https://bit.ly/3GYP4hz
- https://bit.ly/3KOTyrZ
- https://bit.ly/40nq7TF
- https://bit.ly/41ES9ez
- https://bit.ly/41HEjrL
- https://nyti.ms/3Aa2qDR
- https://bit.ly/3ovKlxd
- https://bit.ly/3KS9Gcd
- https://bit.ly/3Aba2Wv
- https://bit.ly/3LeKsX9
- https://bit.ly/3GSWToX
- https://bit.ly/41E1gvY
- https://bit.ly/3LeJDNU
- https://bit.ly/3LdJv0Z
This article was published on August 18, 2023.